How to Calculate Internal Energy: A Clear and Knowledgeable Guide
Calculating internal energy is an important concept in thermodynamics that helps us understand how energy is stored and transferred in a system. Internal energy is the total energy of a system due to the motion and position of its particles, and it includes both the kinetic and potential energy of the particles. Understanding how to calculate internal energy is essential for predicting the behavior of a system and for designing efficient energy systems.
There are several ways to calculate internal energy, depending on the specific system and the information available. One common method is to use the first law of thermodynamics, which states that the change in internal energy of a system is equal to the heat added to the system minus the work done by the system. This equation can be used to calculate the internal energy change of a system during a process, such as a chemical reaction or a change in temperature.
Another method for calculating internal energy is to use the ideal gas law, which relates the pressure, volume, and temperature of a gas to its internal energy. This equation can be used to calculate the internal energy of a gas, as long as the pressure, volume, and temperature of the gas are known. Other factors that can affect internal energy include the chemical composition of the system, the external pressure and temperature, and the presence of any external fields, such as magnetic or electric fields.
Fundamentals of Internal Energy
Definition of Internal Energy
Internal energy is the sum of all the kinetic and potential energies of the particles that make up a system. It is a state function, which means that it only depends on the current state of the system and not on how the system arrived at that state. The internal energy of a system can be increased by adding heat or doing work on the system.
First Law of Thermodynamics
The first law of thermodynamics is the law of conservation of energy. It states that energy cannot be created or destroyed, only transferred or converted from one form to another. In other words, the total energy of a closed system remains constant. This law is expressed mathematically as:
ΔU = Q - W
Where ΔU is the change in internal energy, Q is the heat added to the system, and W is the work done on the system. If heat is added to the system and no work is done, the internal energy of the system increases. If work is done on the system and no heat is added, the internal energy of the system also increases.
State Functions
Internal energy is a state function, which means that it only depends on the current state of the system and not on how the system arrived at that state. State functions are important because they allow us to calculate changes in a system without knowing the exact path it took to get there. Other examples of state functions include pressure, volume, and temperature.
Overall, understanding the fundamentals of internal energy is essential for calculating changes in a system. By knowing the definition of internal energy, the first law of thermodynamics, and state functions, one can accurately calculate changes in a system's internal energy.
Calculating Internal Energy
Calculating internal energy involves understanding the role of heat and work in a system, as well as the internal energy change (ΔU).
The Role of Heat
Heat is a form of energy that can be transferred between a system and its surroundings. When heat is added to a system, the internal energy of the system increases. Conversely, when heat is removed from a system, the internal energy of the system decreases. The amount of heat transferred is typically denoted as q.
The Role of Work
Work is another form of energy that can be transferred between a system and its surroundings. When work is done on a system, the internal energy of the system increases. Conversely, when work is done by a system, the internal energy of the system decreases. The amount of work done is typically denoted as w.
Internal Energy Change (ΔU)
The internal energy change (ΔU) of a system is the sum of the heat transferred and the work done. This can be expressed as:
ΔU = q + w
This equation is known as the First Law of Thermodynamics, which states that energy cannot be created or destroyed, only transferred or converted from one form to another.
Calculating internal energy can be useful in a variety of fields, including chemistry, physics, and engineering. It can help predict how a system will respond to changes in temperature, pressure, or other variables.
Measurement of Internal Energy
Calorimetry
One way to measure the internal energy of a system is through calorimetry. Calorimetry is the measurement of the heat transferred during a chemical reaction or physical change. By measuring the heat transfer, one can calculate the change in internal energy of the system.
Calorimetry experiments can be conducted at constant volume or at constant pressure. At constant volume, the change in internal energy is equal to the heat transferred to the system. At constant pressure, the change in internal energy is equal to the heat transferred to the system plus the work done by the system on its surroundings.
Pressure-Volume Work
Another way to measure the internal energy of a system is through pressure-volume work. Pressure-volume work is the work done by a system when it expands or contracts against an external pressure. The work done is equal to the product of the external pressure and the change in volume.
The change in internal energy of a system can be calculated using the first law of thermodynamics, massachusetts mortgage calculator which states that the change in internal energy of a system is equal to the heat transferred to the system minus the work done by the system on its surroundings. If the system is at constant volume, the work done is zero and the change in internal energy is equal to the heat transferred to the system. If the system is at constant pressure, the change in internal energy is equal to the heat transferred to the system minus the pressure-volume work done by the system.
Combining calorimetry and pressure-volume work measurements can provide a more accurate measurement of the internal energy of a system. By measuring the heat transferred and the work done, one can calculate the change in internal energy of the system.
Applications of Internal Energy Calculations
Thermochemical Equations
Thermochemical equations are chemical equations that include the enthalpy change (ΔH) of the reaction. The enthalpy change is the heat absorbed or released during a reaction at constant pressure. By using the enthalpy change and the internal energy change (ΔU), it is possible to calculate the heat capacity of the system. This information is useful in determining the efficiency of a reaction.
Thermochemical equations also help in determining the energy content of a fuel. By knowing the enthalpy change and the internal energy change, the amount of energy that can be obtained from the fuel can be calculated. This information is useful in the development of new fuels and in the optimization of fuel consumption.
Hess's Law
Hess's Law states that the enthalpy change of a reaction is independent of the pathway taken. This means that the enthalpy change of a reaction can be calculated by summing the enthalpy changes of a series of reactions that add up to the overall reaction. Hess's Law is useful in cases where it is difficult or impossible to measure the enthalpy change directly.
The internal energy change can also be calculated using Hess's Law. By summing the internal energy changes of a series of reactions, the internal energy change of the overall reaction can be calculated. This information is useful in determining the energy content of a fuel and in the optimization of fuel consumption.
Overall, the applications of internal energy calculations are vast and varied. They are useful in a wide range of fields, including chemistry, physics, and engineering. By using these calculations, it is possible to optimize reactions and fuel consumption, and to develop new fuels and materials with improved energy efficiency.
Thermodynamic Processes
Thermodynamics is the study of the relationship between heat, energy, and work. A thermodynamic process is a change in the state of a system that involves the transfer of heat and/or work. There are four types of thermodynamic processes: isothermal, adiabatic, isobaric, and isochoric. Each process is characterized by a specific set of conditions that determine how heat and work are exchanged between the system and its surroundings.
Isothermal Process
An isothermal process is a thermodynamic process that occurs at constant temperature. During an isothermal process, the internal energy of the system remains constant. This means that any heat added to the system is used to perform work, and any work done on the system is converted into heat. An example of an isothermal process is the expansion of a gas in a cylinder with a movable piston.
Adiabatic Process
An adiabatic process is a thermodynamic process that occurs without the transfer of heat between the system and its surroundings. During an adiabatic process, the internal energy of the system changes due to work done on or by the system. An example of an adiabatic process is the compression of a gas in a cylinder with a fixed piston.
Isobaric Process
An isobaric process is a thermodynamic process that occurs at constant pressure. During an isobaric process, the system can exchange heat and work with its surroundings. An example of an isobaric process is the heating of a gas in a container with a constant volume.
Isochoric Process
An isochoric process is a thermodynamic process that occurs at constant volume. During an isochoric process, the system cannot exchange work with its surroundings, but it can exchange heat. An example of an isochoric process is the heating of a gas in a container with a fixed volume.
Understanding the different types of thermodynamic processes is essential for calculating internal energy. The internal energy of a system is determined by the initial and final states of the system, as well as the type of thermodynamic process that occurs between those states. By applying the first law of thermodynamics, which states that the change in internal energy of a system is equal to the heat added to the system minus the work done by the system, one can calculate the internal energy of a system for any given process.
Practical Considerations in Thermodynamics
Path Dependence
The calculation of internal energy can be path-dependent, meaning that the path taken to reach a particular state can affect the internal energy of the system. This is because the internal energy of a system is a state function, meaning that it depends only on the current state of the system and not on how it got there. However, the path taken to reach that state can affect the amount of work done on or by the system, which can in turn affect the internal energy.
For example, consider a gas that expands from an initial volume of V1 to a final volume of V2. The work done by the gas is given by the integral of the pressure with respect to volume over the path taken from V1 to V2. If the gas expands slowly and reversibly, the pressure can be assumed to be constant, and the work done can be calculated using the equation W = -PΔV. However, if the gas expands quickly and irreversibly, the pressure cannot be assumed to be constant, and the work done cannot be calculated using this equation. In this case, the path taken to reach the final state affects the work done and, therefore, the internal energy of the system.
Reversible vs. Irreversible Processes
The calculation of internal energy also depends on whether the process is reversible or irreversible. In a reversible process, the system can be returned to its initial state by reversing the process, and the work done on or by the system is equal to the maximum work that could be done in a reversible process. In an irreversible process, the system cannot be returned to its initial state by reversing the process, and the work done on or by the system is less than the maximum work that could be done in a reversible process.
For example, consider a gas that is compressed from an initial volume of V1 to a final volume of V2. If the compression is done reversibly, the work done on the gas is equal to the area under the pressure-volume curve on a graph of pressure vs. volume. If the compression is done irreversibly, the work done on the gas is less than the area under the pressure-volume curve. In both cases, the internal energy of the gas changes, but the amount of work done on the gas and, therefore, the amount of change in internal energy, is different for reversible and irreversible processes.
In summary, the calculation of internal energy is affected by the path taken to reach a particular state and whether the process is reversible or irreversible. It is important to consider these practical considerations in thermodynamics when calculating internal energy to ensure accurate results.
Frequently Asked Questions
What is the formula for calculating the change in internal energy?
The change in internal energy can be calculated using the formula ΔU = Q - W, where ΔU represents the change in internal energy, Q represents the heat added to the system, and W represents the work done by the system. This formula is based on the First Law of Thermodynamics, which states that energy cannot be created or destroyed, only transferred or converted from one form to another.
How can you determine the internal energy of a gas using temperature?
The internal energy of a gas can be determined using the formula U = (3/2) nRT, where U represents the internal energy, n represents the number of moles of gas, R represents the gas constant, and T represents the temperature in Kelvin. This formula is based on the kinetic theory of gases, which states that the internal energy of a gas is related to the average kinetic energy of its molecules.
What is the relationship between pressure, volume, and internal energy?
The relationship between pressure, volume, and internal energy is described by the First Law of Thermodynamics. According to this law, the internal energy of a system is equal to the sum of the work done on the system and the heat added to the system. When a gas is compressed, work is done on the gas, which increases its internal energy. Conversely, when a gas expands, work is done by the gas, which decreases its internal energy.
How is heat related to the internal energy of a system?
Heat is related to the internal energy of a system because it represents the transfer of energy from one object to another due to a temperature difference. When heat is added to a system, the internal energy of the system increases. Conversely, when heat is removed from a system, the internal energy of the system decreases.
What methods are used to measure the total internal energy of a system?
There are several methods used to measure the total internal energy of a system, including calorimetry, which involves measuring the heat exchanged between the system and its surroundings, and thermodynamic calculations, which involve using equations and formulas to calculate the change in internal energy.
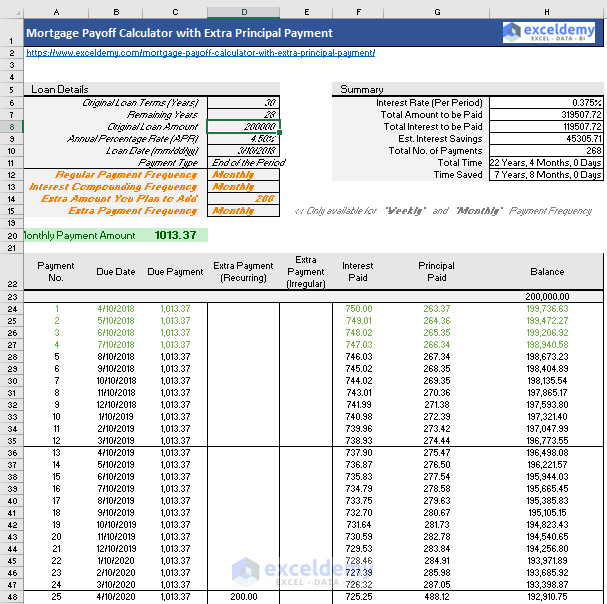
How do you calculate specific internal energy using a formula?
The specific internal energy of a substance can be calculated using the formula u = U/m, where u represents the specific internal energy, U represents the total internal energy, and m represents the mass of the substance. This formula is useful for determining the internal energy per unit mass of a substance, which can be used to compare the internal energies of different substances.